Flash and JavaScript are required for this feature.
Download the video from Internet Archive.
Description
Beginning with the fight or flight response, this Halloween lecture looks in more detail at cellular signaling pathways in action.
Instructor: Barbara Imperiali
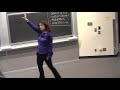
Lecture 21: Cell Signaling ...
PROFESSOR: OK, here we go. Couple of things. Sorry, I forgot to bring candy, but it'll be on sale next week. So we can probably bring it next week. But walking over, I thought, boy, those of you who are here deserve some candy.
But I've just tried to sprinkle in a few interesting slides for your benefit. I saw this on the MIT news of the day, and I thought that was really cool. Who would have thought to turn the giant dome into a Halloween pumpkin?
And I was also jealous this morning when my husband got ready to go work in the emergency room and he put on his Star Trek outfit. So I was like, oh, I didn't even have a-- because I don't usually get to actually have a class on the day of Halloween. So he headed off. I think people are unfortunately going to expect him to be able to really fix things very readily in the emergency room today because he's going to have all those extra powers that he doesn't usually have.
But anyway, so actually it's kind of a good day for a lecture, Halloween, because we're going to talk about the fight or flight response, which is a great paradigm for cellular signaling. So you're going to see how signaling really works in action. Because what one has to think about with respect to cellular signaling is that it's dynamic and transient.
And when we look at the molecular details of the switches that enable dynamics and transient behavior in cells, you're going to see how perfectly adapted they are for these types of responses that have to be carried out in cells or in organs in order to respond to a particular signal rapidly and with a definitive time frame and then have that signal then stop once the time frame has passed. So I really want to sort of stress to you the characteristics of signaling that can emerge just by knowing about two particular cellular switches, knowing the molecular details of those switches, to really understand, then, when we look at them in action in a couple of cellular signaling pathways, then we'll see how adapted those signals are.
And the great thing about biology is that once you learn a few very specific things, then those often get reused in nature. So the cellular signals that I'm going to describe to you are used again and again in different formats to create different signaling pathways. So it's not at every pathway. And every cell in the body has different nuances. It has general paradigms that we can learn about and understand, all right? So the key feature is then to think about the molecular basis of these switches.
So last time, I was talking to you about cellular signaling. And remember, there's always a signal, a response, and an output. So something happens. It's a signal. It's usually a molecule of some kind outside the cell or able to diffuse into the cell.
As a function of that signal, there's a response. So this is molecular. The response obviously is biochemical. And the output is biological.
So I want you to sort of think about these as we look at pathways. What's really my output at the end of a particular signaling pathway? What was my input? How did it get to the cell? How does it have a dramatic effect on the cell as a whole? How does the timing and action of this effect occur so rapidly?
So we talked last time about different types of signals, those that mostly occur in the cytoplasm of the cell with signals that are able to diffuse across the plasma membrane and bind to an intracellular receptor and then cause an action. But really, the most important ones for today are going to be the types of receptors that span the membrane. And the reason why these are much more significant, they're more in number, they're more predominant, is if you have a membrane spanning receptor, you have the opportunity to use signals of very, very different types. Small polar molecules, small proteins, lipids, amino acids, carbohydrates.
You've got a much larger range of signals than you could possibly have if you restricted yourselves to the type of signals that can cross the cell membrane. Those are very limited to non-polar small molecules that can get across the membrane. The more dominant types of signals are going to be the ones that are outside the cell. They arrive at a cell surface. They bind to a receptor that is transmembrane and transduce a signal from the outside to the inside. So that's an important term here, the process of transducing external information to internal information.
So when we started the course, we were really thinking about laying down the cellular membrane as an encased environment where things could occur at high concentrations. You could set up systems that were functional within membranes. But in doing that within a membrane encased area, in doing that, you've built a formidable barrier around the cell. So the types of receptors that we'll talk about are those that have adapted to take this external information into the cell and then have a cellular consequence occur.
So I'm going to talk to you about two specific types of cellular switches, and they're going to be intracellular. And we're going to be referring back to these because they're going to be important as we dissect a signaling pathway. So the first type-- so stand aside a moment. Put aside a moment the actual process of a signal binding the response, both biochemical and biological. Let's look first at the molecular detail of these switches and see how they are adapted to their function.
And the first type of cellular switch are what are known as G proteins. The G is because they bind guanidine nucleotides. So that's why they're called G proteins. They're small proteins that bind GDP or GTP. So this is the guanine nucleotide that has either two phosphates, guanidine diphosphate or triphosphate.
So there may or may not be a third phosphate here. And the G proteins bind them. And the dynamics of the situation are that when the G proteins are bound to GDP, they are inactive. The switch is off.
There's an aspect of the structure, and it's very dependent on how many phosphates there are in this structure. But it's when it's bound to the diphosphate variant of the nucleotide, then it's an off switch. And when it's bound to GTP, it's an on switch and it's active. So this is the molecular basis of one of the switches.
It relies on the shape, the conformational dynamics of these small G proteins. And that shape is quite different if it's bound to guanidine diphosphate or triphosphate. And we'll take a look in a moment at how the structure, the shape, changes. The shape shifts upon binding the triphosphate analog.
So this is a dynamic interconversion. When the GTP is hydrolyzed, you go back to the GDP-bound state, the off state. And there are a variety of proteins that actually help these processes, which we won't talk about in any detail. The main thing that you want to remember is that when the G proteins are bound to GTP, they're in a non-state. GDP, they're in an off state. And that's shown in this cartoon.
And here I should be able to, if all goes according to plan, show you the structure of a GTP analog bound to a G protein. So lets-- this little guy is twirling around. He's settled down a little bit.
The key thing you want to look at is where it's magenta and cyan, the structure of the GDP and GTP-bound G protein are very, very similar. But big changes happen in the yellow, which is the GDP-bound form, and the red, which is the GT-bound form. Let me go back again so you can see that one more time.
So in the GTP-bound form, a portion of the protein swings around and binds to that third phosphate on the GTP and forms a different shape to the structure. And that's a dynamic change that's responsible for activation. When it's just GDP, that's shorter. There's nothing for that red arm to bind. And so it's much more of a floppy structure.
What I want you to notice in that little yellow portion in the GDP-bound form, you actually don't really see where the rest of the protein is. This is because this is a crystal structure. And in the crystal structure, when things are very mobile, you can't even see electron density. It's as if the part of the protein isn't there because it is so dynamic. It's only in the GTP-bound form it forms this tight, compact structure that represents a switch that has been turned on.
Does that make sense to everybody? Is everyone good with that? So just that change, that extra phosphate reaching further to the protein and making an interaction with the protein itself, makes the difference in the dynamics of the G protein and the activity here.
Now, there are different types of G proteins. And you'll see both types reflected in this lecture. There are small G proteins, and they are monomeric.
And then there are slightly more complicated G proteins that are trimeric. They have a heterotrimeric, structure. So they have quaternary structure where you have three different proteins as part of the complex. So the other ones are trimeric. And the G protein actually comprises three subunits where one of them is the important one that binds GDP or GTP.
But they are a little bit more complicated. And in the first example when I talk about a particular response to adrenaline, we're going to see the trimeric G proteins. And because they're trimeric, that means there's three subunits.
And the convention is that they get the Greek lettering system. So they are the alpha, beta, and gamma subunits. They've each got their name. They're three independent polypeptide chains. And it's actually the alpha subunit that binds GDP or GTP. So that's the formulation of one of the types of switches that we're going to see when we start to look at a pathway.
What do you need to remember here, you need to focus on the fact that in one state, the protein is in an off state. It doesn't kick off a signaling pathway. But in the other state, the protein is a different shape because of binding a loop-- let's just make this a little longer-- to that phosphate that's negatively charged to the protein. So that's an on state.
And they are very definitive types of structures. Now, both of these proteins are intracellular, which means they're part of the response once a signal reaches a cell. They are things that change. And they're what the signal gets transduced to, the G proteins.
Now, there's another type of intracellular switch which is used very, very frequently in nature. And in fact, it crosses, permeates, through all kinds of cellular processes. And this is phosphorylation. So here are the G proteins, which is one. And the other one is phosphorylation. I don't like that chalk.
OK, now remember, we talked about reactions of proteins that alter their behavior, their properties, their dynamics. So protein phosphorylation, remember, is a post-translational modification, a PTM. It's something that happens to a protein after it has been translated and folded.
And the PTMs in a phosphorylation involve amino acids that have OH groups. So that's the structure of tyrosine where the squiggles represent the rest of the protein. And on phosphorylation, we append a phosphate group-- whoops, a minus, a minus-- to the oxygen on tyrosine on the side chain.
So actually, it looks pretty different. It behaves pretty differently. There are two other residues in eukaryotic cells that are commonly phosphorylated.
There are the other two that include OH groups. So they are serine and the third one-- as I run out of space, but you get the general message-- and threonine. So these are the three amino acids that commonly get a phosphate group attached. And they change their properties.
They are called kinases. Kinase, the root of the word is actually "to change." So the enzymes that catalyze this change are known as kinases. And in contrast to the G proteins that use GTP, the kinases most commonly use ATP to give up a phosphate to phosphorylate the protein. So there's another substrate in this reaction is ATP.
Now, when we look at this structure, there's two or three things I really want to call your attention to. If we're dealing with this kind of switch, we can go back to the off state by chopping up the GTP and making it GDP again. So that's how to turn the light back off.
In the case of the kinases, we've got to do something to go back from this state to the non-modified state to turn the light switch off. So for every transformation in the cell that involves kinase, there is a corresponding set of enzymes that reverse the reaction called a phosphatase. It takes that group off again.
So let me write that down here. Now, phosphate is used a lot. So this is a phosphoprotein phosphatase. So kinase puts the phosphate on. The phosphoprotein phosphatase takes the phosphate off.
There are three types of amino acids that get most commonly modified in our cells-- tyrosine, serine, and threonine. And one of the ones that forms an important part of an extracellular signaling mechanism are the tyrosine kinases. And we'll delve into them in a little bit, not the section I'm going to cover now, but later. Because tyrosine, various kinases come in a lot of different flavors. The common flavors are whether you modify tyrosine or threonine/serine, because these are more similar to each other, and this guy is different. But we'll get to that later.
So in the cell, we have about 20,000 genes that encode proteins, encoding genes. All right, 515 of those are kinases. That's a pretty big chunk of the genome you've got to accept. So in excess of 500 kinases.
Specifically, protein kinases, the ones that modify. So there's a big hunk of the genome dedicated to this kind of activity. And there's a dynamic because there's also about 100 phosphatases. They are a little bit more promiscuous. You don't need so many of them.
But a large sort of component of the genome is responsible for phosphorylating proteins and dephosphorylating phosphoproteins. And that part of the genome has its own special name. And it is called the kinome. I hope that's not too small in there.
So if we're describing all the enzymes in the genome that catalyze phosphorylation, we would call it the kinome as a collective set. Because it's the set of kinases. And you'll hear that term quite commonly. And the kinome is really important and represents major, major therapeutic targets. Because it's when kinases go wrong that we have physiological defects.
So let's just go back to this. You can see we've got the kinase and the phosphatase. The donor for phosphorylation is ATP. And it is the gamma phosphate that's transferred to the protein to switch it from the off state to the on state. It is a post-translational modification, meaning it occurs on a protein after the protein has been fully translated.
There are a few cotranslational PTMs. That seems like a bit of an oxymoron, cotranslational modifications. But phosphorylation isn't one of them. Glycosylation is. And we won't go into those in any detail even though it breaks my heart not to go into those.
But OK, all right, so now I want to first of all introduce you to a paradigm for signaling as opposed to really go into what's happening. And so one of the first paradigms is a situation where you have a cell. In the plasma membrane of that cell is a receptor. And it gets hit with a signal. So a signaling paradigm is that a molecule from outside the cell binds to something that's transmembrane.
And then you start getting signal transduction through a pathway. So any extracellular signal could be game in this process. And then there's a sequence of events. Upon signal binding, there's a sequence of changes that ends you up with a final output. And generally, signaling pathways go through a number of steps where there is the opportunity for the amplification of a signal.
So I talked to you last about time about some of the hallmarks of signaling. Specificity defines how accurately that extracellular signal binds to the receptor. But amplification really refers to how signals get bigger and bigger through certain steps in a signaling pathway in order to have a big impact in the cell, not just a single event going through a single pathway one molecule at a time. And we'll see that in the example that I show you.
And then oftentimes when we look at signaling pathways, we care a great deal about what's the first response upon the signal hitting the outside of the cell. So in many signaling pathways, this could be a protein. The receptor could be a protein that is bound to a G protein. And that would be the first responder through the pathway that really triggers off the cellular events.
OK, and now, so what I want to talk about now, is the-- I always get this wrong. I always thought it was flight or fight or fight or whatever, fright. I always thought it was flight or fright, but it's not. The response is actually the fight or flight.
So let's set this up to understand why this is such a great manifestation of a cellular signaling response. Because it includes a lot of the hallmarks that are really characteristic of the cellular response. So this response involves a cellular receptor that is called a G protein coupled receptor. We saw a little bit about them last time.
They are always called GPCRs for short. And what that term means is that it's a receptor that is linked in some way to a G protein. So it could be coupled to a monomeric or a trimeric G protein.
So don't confuse the two. One is the receptor. It's transmembrane. It's responsible for receiving signals and transducing them. The first responder is the G protein that changes from a GDP-bound stage to a GTP-bound state. And in the one that we're going to talk about, we're going to deal with a trimeric G protein in the fight or flight response.
And what you see here is a cartoon of the players that are involved. So remember the G proteins? We talked about them briefly last time.
They have seven transmembrane helices. They span the membrane. They have the N terminal outside. 1, 2, 3, 4, 5, 6, 7. N terminus out, C terminus in. And each of these is a transmembrane helix. And this would be outside the cell. This would be in the cytoplasm.
OK, and you can actually often look at the transmembrane protein and know its behavior. Because the width of these transmembrane helices often comes in at approximately 40 angstrom, which is the span of a membrane. You can sort of say, that looks like a transmembrane helix because it's exactly that dimension to cross a membrane. And the GPCRs in this case would bind to a ligand outside the cell and have a response inside the cell. So those seven transmembrane helices are responding to ligand binding.
So let's take a look at this picture because it's almost impossible for me to get it onto the screen. So it binds to a trimeric G protein. Remember, I talked to you about the two different types. The trimeric has an alpha, beta, and gamma subunit quaternary structure.
And they're shown here in different colors. The green is the alpha subunit. The red is the gamma subunit. And the yellow is the beta subunit. So what happens, when the ligand binds the G protein coupled receptor, there is a reorganization of those seven transmembrane helices. Last time, I identified them to you.
When you look at a couple of these, they're actually fairly large loops that grab onto your ligand. And then that will translate conformational information through the membrane to the other side where the G proteins are sitting. And in this response, what happens is upon binding the ligand, the alpha subunit leaves the team and goes from their GDP-bound state to the GT-bound state. So it literally changes its state and changes its mode of association within the cell upon that action.
So you can see how nicely we have transduced the ligand binding out here to a pretty discrete cellular event, turning on the switch of the G protein alpha subunit. Is everyone following me there? I know it sort of looks complicated to start with. But you'll see it in action.
OK, so here are the cellular components of the response. So basically, this is the kind of response where if you get scared or you feel you're in harm's way, you will trigger this response in order to generate a lot of ATP in order that you can respond-- run away, hide, do something very active in order to rapidly respond to a threat of some kind. And this response is triggered by a small molecule. In this case, it's epinephrine or adrenaline. Different names on different sides of the Atlantic, but you all know what adrenaline is.
And here's the structure of epinephrine or adrenaline. And it is the signal for the flight or fight response because it's the small molecule that binds to the extracellular surface of the receptor, changes its shape so that things can happen intracellularly. And it's just one small molecule. Normally that would be charged. It doesn't diffuse across the membrane. So it's stuck being on the outside of the membrane, OK?
So this is the signal that triggers the response. So if you have to respond to a threat of some, kind you can't stop, sort of go to the fridge, get a big snack, eat it, digest all your food, and hope you're going to get energy quickly. What you've got to do is have a response where you can generate energy from your glycogen stores that are in the liver. So there is a signal comes from the adrenal region, which is the release of adrenaline that goes to the cell surface receptors to trigger the response.
And what kind of signal would this be? Would it be paracrine, autocrine, exocrine? What kind of signal would that be? Sorry-- endocrine, paracrine, juxtacrine Do you remember last time? Yeah--
AUDIENCE: Endocrine.
PROFESSOR: Endocrine, so it's a response that comes from the kidneys and goes to the liver. So it's going, it's traveling. Autocrine is self. Paracrine is near. Juxtacrine is cell contact. But any of these hormonal responses are pretty commonly endocrine responses.
So what happens once the signal binds? So the specificity in this situation is that the G protein coupled receptor-- now shown in pink in very stylized form, but you can count those seven transmembrane domains-- will bind exclusively to this GPCR with high specificity. Another signal, another small molecule that looks like it won't bind because we have to have specificity for the signal.
Upon that binding event, it will trigger a change within the cell. And that change within the cell is that the alpha subunit-- here you see alpha subunit, beta, gamma. They're all shown in green. The alpha subunit of the G protein, remember, I told you it was a trimeric G protein where the alpha subunit is the key player. The alpha subunit leaves the team and it exchanges its GDP-- that's its resting state, nothing's happening-- for GTP, which turns it on.
So that's the first response. The G protein is responding to the signal from the outside of the cell through the auspices of the G protein coupled receptor to give a change within the cell that's a discrete change. OK, following me so far?
So now what we need to do is trigger the remaining biochemical events that are going to get us out of this sticky situation where we need to produce a lot of ATP. So it turns out that the GTP-bound form of the alpha subunit can then bind to another enzyme. And that enzyme is adenylate cyclase. So we've bound, we've changed the GDP to GTP, there's a response, and we activate the enzyme known as AC, which you look up here, it's called adenylate cyclase.
So this is a messenger within the cell that's now being generated as a response to the signal coming from outside through the GPCR to the alpha subunit of the G protein, which, then, in its GTP-bound state, binds adenylate cyclase. OK, is everyone with me? And once that is bound, adenylate cyclase can do its biochemistry. And the biochemistry that adenylate cyclase does is shown down here.
Here's ATP. Adenylate cyclase cyclizes ATP. You lose two of the phosphates, and you get this molecule known as cyclic AMP, which is a messenger molecule that will propagate information through the cell. So now, the adenylate cyclase is activated because it's bound to the GTP-bound form of the alpha subunit.
That means we can make a bunch of cyclic AMP. And cyclic AMP is what's known as a second messenger. And that often means it's a common messenger in a lot of pathways. It shows up quite frequently within the wiring of a pathway. And it acts locally to where the pathway is being processed.
So once cyclic AMP is formed by adenylate cyclase, that then activates an enzyme. It activates protein kinase A. So PKA is a kinase. It's actually a serine threonine kinase. And that then results in certain proteins within the cell becoming phosphorylated to continue propagating our effect.
So we have specificity by the adrenaline binding. We have amplification somewhere in this pathway. So I told you that many pathways go through steps where you start amplifying the signal.
Where do you think is the first stage in this set of transformations that I've described to you where you start amplifying the information? Think about what each of the events comprises. Is this one binding to one in one event, or is it one binding to one and we get multiple events?
Where is the first step of amplification that's essential? Because it wouldn't do us any good if we make one molecule of ATP at the end of the day. We've got to make dozens and dozens of molecules of ATP. What's the first event that could be an amplification? Over there--
AUDIENCE: Is it when [INAUDIBLE]
PROFESSOR: Yeah, when it's made, yes. So this, let's go through them. One binds to one, great. Once one binds to one, one of these is released. It gets converted to one of these. Once one of these is made, adenylate cyclase is an enzyme, so it can make a bunch of cyclic AMP, which can then activate a bunch of protein kinase A, which can then phosphorylate a bunch of cellular proteins.
So we've got an expansion of our response. All right, so everyone, does that make sense to everyone? So amplification is really important. Feedback is also important.
If you ended up needing an EpiPen because you have an allergic response, you might remember that you've got the jitters forever because there's too much firing and action going on. But in the fight or flight response, there's feedback at a certain stage that slows down this entire process. And that feedback actually comes from an enzyme that chomps up the cyclic AMP to make it inactive as a second messenger. So there's feedback in this process.
OK, now, what happens within the cell to get us that biological response? This is a sort of a shortened version of what's happening. So epinephrine binds. Here we are with the alpha subunit with cyclic AMP. For each one of these, you might make 20 molecules of cyclic AMP. That would inactivate many, many PKAs.
And then you go through a series of biochemical steps where different enzymes are activated with the overall goal of in the liver chewing up glycogen. OK, so glycogen is a pretty impenetrable polymer of carbohydrates. And you need several enzymes to start to break glycogen down to make glucose phosphate. And so these enzymes here of phosphorylase, B kinase, glycogen phosphorylase all end up converting glycogen into glucose 1 phosphate.
So you access your liver stores of stored carbohydrate, which is in a polymeric form, to get a lot of glucose phosphate, which is then hydrolyzed to glucose, which then hits the blood system. And then you can deliver glucose to all the cells to go glycolysis and make ATP. And every glucose molecule, as you know, can really churn out ATP.
So what we see in this process is going through the entire dynamics of the system where we've seen specificity, amplification, and feedback. Later on, I'll describe integration to you. OK, everyone following? The series of steps that go from a molecular messenger to biochemical steps to physiological, biological response.
Now, I want to just emphasize one quick thing here. I've got a couple of slides I popped in of drug targets. About 45% are receptors in cells. 25% of the entire drug targets are GPCRs. They respond to all kinds of signals-- amines, amino acids, lipids, little peptides, proteins, nucleotides-- all commonly going through the G protein coupled receptor to give you a similar phenomenon to what I've described to you.
And what I think is particularly interesting-- I'm going to post all of these as slides. What I want you to see, this was quite a while back, but it just shows you so many of trademarked drugs that target different GPCRs-- they're shown here-- and what diseases they're used for to treat, and what's the generic name of those drugs. So you can see here many, many diseases have at the heart and soul of their problem different receptors. And these are all G protein coupled receptors that are treated with small molecules that bind to the receptor and often glue it in an inactive state so it can't, then, bind to an activating signal and have all the rest of the events occur.
There are very few structures of the G protein coupled receptors, but there are some of them. So many of the target G protein coupled receptors can be modeled computationally. And then you can do a lot of work where you actually model the receptor in a membrane environment and start searching for drugs through computational approaches. And I thought a lot of you might be interested in this. Because this is a really strong axis where bioinformatics, confirmation, and advanced physics and molecular dynamics can be brought to bear on drug discovery when you don't have perfect molecular models of your targets.
OK, so now we're going to move to a different kind of signal. We're going to talk about the receptor tyrosine kinases. All right, so in the receptor tyrosine kinase responses, we can often see very similar paradigms to what I've just shown you. But there is an important distinction. Receptor tyrosine kinases, we often call these RTKs. So that's their shorthand.
So over here, I described to you different kinases. That we have kinases that modify threonine, serine, and tyrosine. The receptor tyrosine kinase is a subset of tyrosine kinases that form part of a receptor. So if you were to think about various kinases, you would have the serine/threonine, and you would have the tyrosine ones.
But these would be differentiated into the ones that are part of a membrane protein, the RTKs, and then the ones that are soluble in the cytoplasm. And the serine/threonine ones are most commonly soluble in the cytoplasm. I'm going to focus on the receptor tyrosine kinases because they do slightly different activities when they signal relative to the GPCRs.
So that's once again, this is a situation, another paradigm where you see a series of events. But with a number of the receptor tyrosine kinase pathways, the ultimate action ends up being in the nucleus where, as a result of an extracellular signal, you get a series of events that ends up with a protein being sent into the nucleus. And that protein may be a transcription factor that binds to a promoter region.
And as a result of that, you'll get gene transcription occur. You'll transcribe a gene, make a messenger RNA that will leave the nucleus and cause action within the cell. So this is a little bit different than the other response that was mainly cytoplasmic.
OK, so let's take a look at the receptor tyrosine kinases. Receptor tyrosine kinases are proteins that span the membrane but rather differently from the GPCRs. And they have a domain that's extracellular, just a single transmembrane domain. So this is out. This is in. And then they have an intracellular domain.
This would be where the ligand binds. This would be how there's some kind of signal transducing. And this would be a kinase domain.
OK, so how do we get the information in? When we saw the GPCRs, we saw the ability of those seven TMs to kind of reorganize and send information in the cell. With the receptor tyrosine kinases, it's kind of different. There are regions of the membrane where there are a lot of these proteins.
They commonly bind small peptides and protein molecules. And when they're in their activated form, once the small protein binds, the receptor tyrosine kinase forms a dimeric structure. That is, two of these get together only upon ligand binding. They move together once there is a ligand bound. And then what happens is that the tyrosine kinase domains phosphorylate each other. And that's activation in the case of receptor tyrosine kinases.
So when the small protein ligand is not around, this is a singleton. It doesn't work on itself. Once this ligand binds, interactions change. You get a dimeric structure where one kinase can phosphorylate what's called in trans the other kinase domains. So it's different from the GPCRs. It's got a different kind of feel to it, but it's still a dynamic transient signal.
Let's take a look at this within a cell and see what kinds of responses-- and this is in response to EGF, which is epidermal growth factor. It's a cytokine that promotes cell division. So a lot happens with respect to the action of a cell, not to produce ATP, but now to respond by producing all the elements that enable cells to grow and proliferate.
So the epidermal growth factor binds. You get dimerization. Upon that dimerization, the kinase domain in one structure-- in the blue one-- phosphorylates the other, and vice versa. They phosphorylate each other intermolecularly.
Once that has happened, through the auspices of another protein I won't bother you with the name of, this phosphorylated intracellular RTK binds to a small G protein. In this case, it's a monomeric G protein, not one of the trimeric ones, a small one known as RAS. Once that binding event occurs, guess what? RAS gets activated. It's now binding GTP instead of GDP.
And then it starts going through a sequence of events where there's a ton of controlled cellular phosphorylation events that result in moving a protein into the nucleus that helps form a transcription complex that results in cellular proliferation. Similar but different series of events. There's still amplification. There's still dynamics. And in this case, it's a lot of phosphorylation events.
And what I want to sort of define for you is that many of these pathways are in trouble in disease states. Be it inflammation, neurodegeneration, or cancer, there is aberrant behavior of proteins within these pathways that cause them to go wrong, cause cells to proliferate out of control or undergo bad responses. And that is why these proteins end up being therapeutic targets like the G protein coupled receptors.
OK, so we've seen the characteristics of signaling. We've seen a signal. We've seen amplification. We've seen responses.
What I just want to quickly show you is an idea about integration. So here's an idea with two signaling pathways that sort of end up with the same signal outside where you integrate actions through two different signaling pathways to achieve a bigger, different kind of response. So that's that last hallmark of signaling pathways. It's not that every pathway is clean and straight. It has cross-talk with other pathways and you get amplified or different responses. Tremendously complicated.
I want to give you one more term. And then I'll show one table. When these pathways go wrong, it's often because switches get stuck on.
So for example, a G protein gets stuck in its GTP-bound state or doesn't even need GTP to be activated. Or a tyrosine kinase is stuck activated. And that's what's called constitutively active, basically meaning it's permanently on. So many of the diseases that are caused by mutations in your genome, not genetic diseases but mutations in your genes in some particular cells, end up with constitutive activation where you don't need a signal to have a response.
And so for example, cells may proliferate out of control. So that is an important term to know and understand. Because constitutive activation basically means that a receptor may be active in the absence of a ligand.
And I believe this is my last slide. I just wanted to leave you with this. When one thinks of GPCRs, there are tremendous therapeutic targets. The world of kinases is no less important. This scale is in billions of dollars spent on developing molecules that may be curative of diseases that involve dysregulated signaling.
And what you see on this, I want to point out two things. Of course this thing stops working at the last minutes. But what I want to point out is this particular bar. This represents the billions of dollars spent on protein kinase inhibitors over a five-year period. And it's just escalating and escalating.
Similarly, monoclonal antibodies are very important. But the small molecule drugs hold a real dominance.
What do these drugs do? They enable you to have small molecules that can go into dysregulated signaling pathways and stop the activity somewhere in the pathway to avoid signals going constantly to the nucleus and turning things on all the time. So both of these types of functions in cellular signaling are ones you want to understand both from a biological perspective but from a medical perspective. OK--